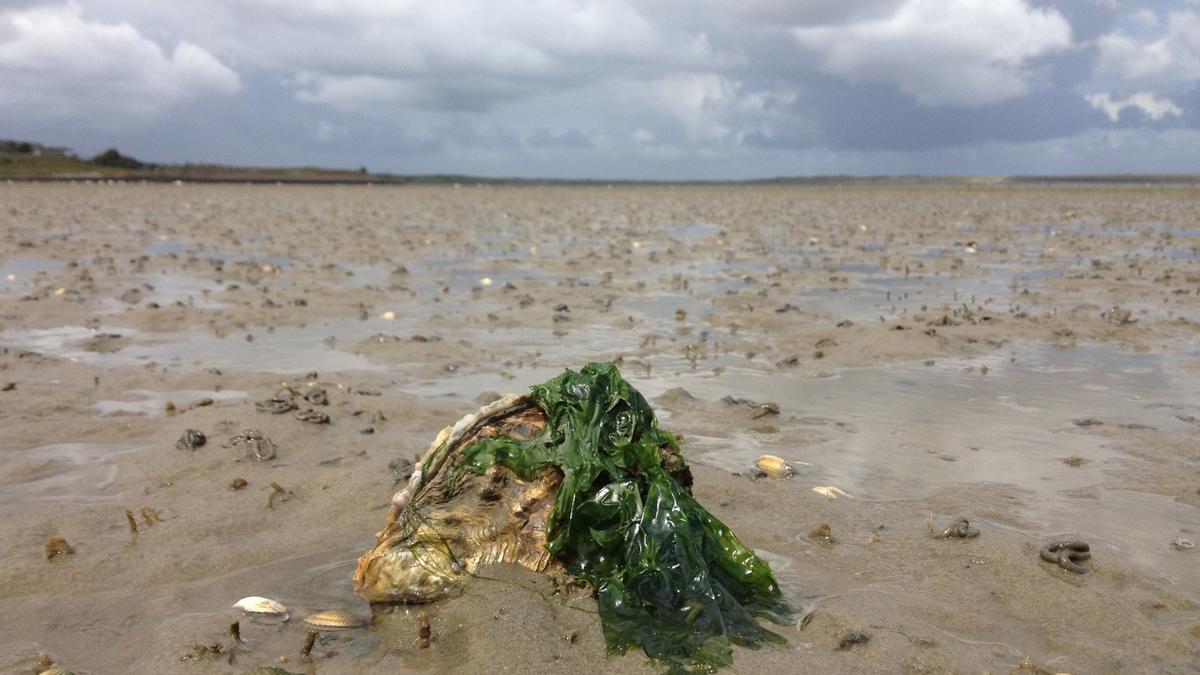
Photo: C.J.M. Philippart. Oyster near Texel.
Climate ecosystems
C.J.M. Philippart, L. Mekkes, C. Buschbaum, K.M. Wegner, K. Laursen
PDF1. Introduction
The inscription of the Wadden Sea as a World Heritage was based on meeting three out of four natural heritage selection criteria, referring to its dynamic landscape (criterion xiii), the undisturbed ecological processes (criterion ix) and the important habitats for species’ conservation (criterion x). The Statement of Outstanding Universal Value (OUV) of the Wadden Sea incorporates EU directives (Habitats & Birds Directive, Water Framework Directive, Marine Strategy Framework Directive) and the guiding principle as set by the Trilateral Wadden Sea Cooperation in 1978, which is “to achieve, as far as possible, a natural and sustainable ecosystem in which natural processes proceed in an undisturbed way” (CWSS, 2010).
The Wadden Sea is presently affected by changes due to climate change. Numerous effects include temperature increase, sea level change, erosion or sedimentation (IPCC, 2007). They will manifest themselves through a number of present-day and anticipated effects of altered seasonal patterns and physiology of organisms, which translates into changes in distribution patterns of species, interspecific relationships and changes in the timing of life cycle events, i.e., phenology (e.g., Beukema, 1992, Beukema et al., 1990, 2009, Beukema & Dekker, 2005, Philippart & Epping, 2009, Witte et al., 2010).
The Wadden Sea, as most coastal marine ecosystems, experiences multiple environmental stressors simultaneously that can differ widely in their pathways and strengths of impact. An understanding of all relevant stressors is critical to prioritise conservation actions, including restoration, protection, and management. Yet, scientific concepts and empirical evidence on the separate and cumulative impacts of all relevant stressors is often insufficient to guide such decisions. Climate change, together with other anthropogenic effects, such as large human-made infrastructures, (reduced) eutrophication, fisheries, pollutants and invasive species, will presumably lead to synergistic and/or cumulative effects.
In this report, first the status and trends of direct and indirect impacts of climate change on key elements of the Wadden Sea ecosystem as encapsulated in directives and principles is described. This information on climate change is based upon long-term field observations and climate model projections, which are partly summarized within other reports of this Quality Status Report and are based to a large degree on data collected within the framework of the Trilateral Monitoring Action Plan (TMAP). The spatio-temporal variation in drivers and responses will be explicitly considered. Findings are discussed in the light of possible consequences for the conservation targets of the Wadden Sea, especially focussing on the comparison of the assessments versus the targets.
2. Status and trends
2.1 Direct impacts of climate change
2.1.1 Introduction
Various aspects of changing weather and climate (including temperature, sea level change, wind and rainfall) are expected to affect species and habitats of the Wadden Sea. Because of the variation in characteristics of different areas of the Wadden Sea including tidal basins (such as average winter temperatures, mean tidal range, exposure to prevailing winds and connectivity to rivers), the impacts of local changes in weather and climate on the species and habitats are expected to vary in space and time throughout the Wadden Sea.
In addition, it must be noted that changes in the frequency of rare and unpredictable extreme events (e.g., heat waves and storm surges) may be more influential on habitats and species than trends in climatic means. In particular, if individuals are meeting the boundaries of their distribution ranges in the Wadden Sea as set by environmental conditions such as summer temperatures (e.g., cold-water bivalves) and emersion times (e.g., birds breeding on saltmarshes).
2.1.2 Temperature
Water temperatures are far from homogenous in the Wadden Sea area. Based on the Hellmann index for winter severity (Hellmann, 1917), winters in the Wadden Sea are generally colder in the north-eastern part compared to the south-western part.
The Wadden Sea is bordering the North Sea, which together with the Baltic Sea, represent the two fastest warming large marine ecosystems worldwide (Belkin, 2009). In the western part in the Dutch Marsdiep tidal inlet, Sea Surface Temperature (SST) measurements started in 1860 (Figure 1).
The first 30 years were characterised as a period in which the annual average temperature continually decreased by 1.5 °C (see also Figure 4 in report on "Climate change"). Since about 1890 up until 1990, average temperature varied without a clear trend. Only the last 25 years showed a warming of about 1.5 °C. So far, 2014 has been the warmest year, with an average water temperature of 12.5 °C (Nauw, 2016).
Compared to the present (1981-2010), annual air temperatures in the Netherlands are projected to rise with 1 °C to 2.3 °C by 2050 and 1.3 °C to 3.7 °C around 2085 (KNMI, 2014). For Germany, yearly mean temperatures are projected to increase by 2.0 °C and 4.9 °C (mean 2.9 °C) in 2100 (GKSS Research Station, www.norddeutscher-klimaatlas.de). Not only average but also extreme temperatures in winter and summer are expected to increase (Sterl et al., 2008a, KNMI, 2014). In 2100, for example, maximum temperatures will rise well above 40 °C (Sterl et al., 2008b) and the number of days with frost might decrease by up to 45 days per year (GKSS Research Station, www.norddeutscher-klimaatlas.de).
Some species may profit from such an increase in winter temperature, as mudflats are less likely to freeze. This has a positive effect on the feeding conditions, with an increased availability of polychaetes, for wintering wader populations that are increasingly more abundant in areas with milder temperatures (Maclean et al., 2008). Beukema & Dekker (2011), for example, observed a significantly positive relationship between winter temperature and number of sampling sites occupied at the Balgzand tidal flats by the polychaete worms Lanice conchilega and Nephtys hombergii. As mean winter temperatures are increasing, other benthic species can be negatively affected. For example, after several consecutive mild winters at the beginning of the new century repeated recruitment failure has been observed for mussels (Mytilus edulis) and cockles (Cerastoderma edule) (Strasser et al., 2003, Beukema et al., 2009). Both species constitute an important food source for bivalve eating birds such as oystercatchers and eider ducks.
Figure 1. Long-term field observations on annual means (red dots) of water temperature in the Dutch Marsdiep, the westermost tidal inlet of the Wadden Sea, from 1861 to 2015. The black line indicates the 10-year running average. Since 1860, the seawater temperature of the Marsdiep has been measured daily by hand, at 8am from the dike near the jetty of the NIOZ Royal Netherlands Institute for Sea Research. Since the year 2000, continuous seawater temperature is also being recorded electronically (Van Aken, 2008, Nauw, 2016).
2.1.3 Sea level and tidal ranges
Sea-level rise is expected to accelerate in the future due to global warming (see also report "Climate change"). Field observations suggest that some systems remain stable as sediment import, tidal-flat and salt marsh accretion can keep pace with sea-level rise when it does not exceed 3-6 mm per year (Van der Spek & Beets, 1992, Bartholdy et al., 2010, Madsen et al., 2007, Elias et al., 2012, Suchrow et al., 2012), while other systems might degrade and finally drown (Van Wijnen & Bakker, 2001). Thus, this process is strongly site specific.
When sedimentation rate does not keep up with a rise in sea level, it may induce the landward migration of the species such as seagrass to limits imposed by anthropogenic barriers such as dikes (Valle et al., 2014). If intertidal areas get lost, birds might lose important foraging and resting regions (Munaretto & Klostermann, 2011).
Laursen et al. (2010b) draw attention to the fact that the Wadden Sea regions where negative trends in bird numbers dominate, are characterized by a large tidal amplitude (central Wadden Sea), whereas areas where bird numbers have generally increased are characterized by a small tidal amplitude (the Netherlands and Denmark). The changes in bird numbers were only attributed to species depending on tidal flats, whereas species depending on adjacent salt marshes did not change in numbers.
Within the Wadden Sea, however, a process of drowning following an increase in high-tide levels is to expected to start earlier and proceed faster in tidal basins with lower Mean Tidal Range (MTR) as in central basins with higher MTR (Hofstede, 2015, see also report "Climate change"). This would imply that mobile species might start migrating from more affected to less affected tidal basins within the Wadden Sea.
2.1.4 Wind and hydrodynamic forcing
In addition to temperature-induced changes in sea level, water heights in the Wadden Sea are also influenced by wind conditions in NW Europe. Historical records indicate that 95th as well as 99th percentile wind speeds in the North Sea basin have increased since 1960 (Alexandersson et al., 2000, Weisse et al., 2005, Feser et al., 2015). Model studies on future (2071-2100) wind conditions in the North Sea Basin show an increase in 99th percentile wind conditions for the W and SW and a reduction for those from the N and NW (De Winter et al., 2012).
If wind-driven wave forcing is an important factor limiting habitat suitability, such changes in wind direction may modify the conditions for potential habitats, e.g., intertidal musselbeds. Within the Dutch Wadden Sea, for example, the eastern part is more suitable for mussel bed settlement compared to the western part. This region is limited by wave forcing, and habitat suitability is more sensitive here to changes in the wave climate (Donker et al., 2015).
Changing wind patterns may furthermore influence the occurrence and height of storm surges. Storm surges temporally limit the emersion time of mudflats and, therefore, the accessibility of resources for waders during low tide. In addition, storm surges increase the flooding risk of saltmarshes. This may affect the breeding success of bird species such as oystercatchers when the number of storm surges increases in the breeding season (Van de Pol et al., 2010).
It is hypothesized that the observed changes in wind direction, sea level and temperature could affect the central Wadden Sea most, in particular with regard to sediment composition (Oost et al., 2009, Philippart & Epping, 2009, Laursen et al., 2010b). In the German Wadden Sea, an increase in grain size was related to an increase in hydrodynamic forcing (Bartholomä & Flemming, 2007). A coarsening of the sediment due to increased hydrodynamic forces may have contributed to the observed reduction in benthic invertebrate stocks (Dolch & Reise, 2010).
2.1.5 Precipitation
Within north-western Europe, extreme precipitation amounts are expected increase throughout the year (IPCC, 2013). Subsequent changes in riverine runoff might locally influence various ecosystem processes, including migration of juvenile fish to nurseries (Vinagre et al., 2009), distribution of bivalve larvae (Folmer et al., 2014) and mortality of cockles (Kristensen, 1958).
Furthermore, rainfall-driven changes in estuarine circulation may affect exchange of suspended sediments and organic matter between the North Sea and the Wadden Sea. Hence, primary productivity and carrying capacity of the Wadden Sea can be influenced if changing precipitation patterns result in changes in river outflows (Burchard & Hetland, 2010, Flöser et al., 2011, Van Beusekom et al., 2012, Jung et al., 2017).
2.2 Indirect effects of climate change
2.2.1 Distribution shifts, phenology and body size
Climate change affects different levels of biological organization. If coexisting species have different sensitivities and differential responses to climate change, there will certainly be an emergence of modified communities with novel species combinations and/or changes in the distribution and strength of their interactions (Lurgi et al., 2012). Early studies focused on population and species-level effects, and showed expansions of species to the poles and/or deeper waters following temperatures as climate warms, together with observations of phenological change (Parmesan & Yohe, 2003, Walther, 2010, Chen et al., 2011). More recently, a number of studies have reported shrinking of body size as an effect of climate warming (e.g., Lurgi et al., 2012, Van Gils et al., 2016).
The distributions of marine species responding to climate change appear to move at the same rate as to be expected from changes in mean ocean surface temperatures (Poloczanska et al., 2013). Generally, further warming will drive new warm-water species from the south into northern regions, whilst local cold-water species might be driven out of the area (Beaugrand et al. 2003, Beukema & Dekker, 2011). In the Wadden Sea, distribution shifts have been observed for species within many taxonomic groups in the Wadden Sea (Figure 2). In some cases, however, marine species that are bound to particular temperatures might actually have to migrate in the direction of the equator (Boersma et al., 2016).
Figure 2. Sea surface temperature map shows a selection of warm-water species shifting northwards, whereas cold-water species retreat the Wadden Sea to keep up with the colder waters. sardines, anchovies (Beare et al., 2004), herring (Clupea harengus) (Alheit & Hagen, 1997), spoonbill (Platalea leucorodia) and the Mediterranean gull (Larus cachinnans) (Koffijberg et al., 2015) migrate northwards and enter the Wadden Sea, whereas cod (Engelhard et al. 2014), particular species of zooplankton (Beaugrand, 2004) and polychaetes (Amphictene auricoma) (Hiddink et al., 2015) retreat from the Wadden Sea and shift polewards. Rocky shore snails (Mieszkowska et al., 2005) and Eurasian curlews (Numenius arquata) (Hornman et al., 2011; Laursen and Frikke, 2013) shifted from the UK towards the Wadden Sea, harbour porpoises moved in from Norwegian waters (Hammond et al., 2013) and Eurasian curlews (Numenius arquata) from the UK (Maclean et al., 2008). Common eiders (Somateria mollissima) retreated and shifted more towards Denmark (van Roomen et al., 2012). Drawing by L. Mekkes.
Phenology, or the timing of repeated seasonal activities, such as reproduction and seasonal migration, is highly sensitive to global warming. Although many marine organisms are responding to interannual changes in temperature, there is considerable variability in magnitudes and patterns of responses (Parmesan & Yohe, 2003). Seasonal changes in the timing of biological events for different functional groups are leading to mismatches (Edwards & Richardson, 2004). This could affect metabolic balances, food availability and interactions between predators and preys, and, in turn, affect mortality and reproduction rates (e.g., Philippart et al., 2003, Visser & Both, 2005).
Changes in phenology appear to have caused alterations within the Wadden Sea food web, creating matches and mismatches between interacting species (Figure 3). Species-specific changes in phenology underline that different species (and even different life phases within one species) might be subject to differing environmental constraints and subsequently respond differently to environmental changes during their life.
Figure 3. Possible mismatches in species compositions due to climate change. Top panels: Warmer temperatures during winter causing epibenthic predators to survive, which increase predatory pressure upon bivalves (Beukema, 2009), which in turn result in a decrease in bivalve-eating birds (Camphuysen et al., 2002), while crab-specialists increase in number (Luczack et al., 2013). Bottom panels: Increased water temperatures cause a shift in plankton composition. This causes an increase of grazing by zooplankton on phytoplankton (Wiltshire & Manly, 2004). As a result, cod recruitment reduces and cod stock declines. Drawing by L. Mekkes.
Shrinking of average body sizes is expected to have consequences for trophic interactions (Sheridan & Bickford, 2011, Lurgi et al., 2012). Smaller bill sizes of knots, for example, reduces their access to deeper buried bivalve prey (Van Gils et al., 2016). If migratory fish or birds are concerned, then growth inhibition at nursery or breeding sites might influence survival in other areas, and subsequently affecting overall population sizes (e.g., Genner et al. 2010, Van Gils et al., 2016).
2.2.2 Distribution shifts in plankton, seaweeds and invertebrates
Within the North Sea, the range of warmer water species of plankton has extended northward by 1000 km in only 40 years (e.g., calanoid copepods), and colder species have retreated out of the North Sea (Beaugrand et al., 2003, 2014). Not all species, however, show a northward migration.
Many warm-water rocky top shells (e.g., Gibbula umbilicalis) and barnacles (e.g., Balanus perforatus), which were formerly absent in the Wadden Sea, have spread south from the tip of Scotland along the North Sea coast of the UK (Mieszkowska et al., 2005). Abundances of several species within species groups of jellyfish, decapods and echinoderms have increased as well (Kirby & Beaugrand, 2009, Gibbons & Richardson, 2009), and appear to flourish in these changing temperature-related conditions with consequences on species relationships in both the plankton and benthos.
Milder temperatures may facilitate the establishment of newly introduced warm water species. Anthropogenic introduced species often originate from warmer coastal areas, such as the pacific oyster (Crassostrea gigas), the American slipper limpet (Crepidula fornicata) or the Australian barnacle (Austrominius modestus) (Witte et al. 2010, see also Figure 4 in see also report on "Climate change"). Many immigrating species are settling in mussel and oyster beds being used as habitat and attachment substratum. Examples are the Japanese seaweed (Sargassum muticum), the Asian tunicate (Styela clava) and Asian shore crabs (Hemigrapsus takanoi, Hemigrapsus sanguineus) (Buschbaum et al., 2012).
2.2.3 Distribution shifts in fish
For fish in temperate waters, higher temperatures generally resulted in an introduction of new species from the south and declines in local stocks (Perry et al., 2005, Rindorf & Lewy, 2006). For the Wadden Sea, an overall increase of fish biodiversity was observed as the result of increasing small southerly species, such as sardines and anchovies (Beare et al., 2004), and a decrease in abundance and range of large northerly species, such as Atlantic Cod (Gadus morhua) (Hiddink & Ter Hofstede, 2008, Engelhard et al., 2014).
In the Marsdiep tidal inlet, the biomass of Eelpout (Zoarces viviparous), for which the Wadden Sea forms the southern border of its distribution, has locally decreased, whilst that of sea bass (Dicentrarchus labrax) encountering its northern border in the Wadden Sea, has increased in concordance with increasing water temperatures (Van Walraven et al., 2016).
Next to immigrating species also local species can profit from mild winters. Here, two non-commercial flatfish species (Buglossidium luteum and Arnoglossus laterna) show this trend of increased abundance as a result of their warm-water prevalence and increased survival rate during mild winters (Van Hal et al., 2010).
2.2.4 Distribution shifts in birds
Milder temperatures have caused shifts in migrating species of birds. During the last 30 years, a northeast shift of wader-populations wintering in Europe has been indicated (Maclean et al., 2008). Also, water bird species normally wintering in the south or south-west, shift northwards. This causes an increase of water bird species in the Wadden Sea, such as the Eurasian curlew (Numenius arquata) (Hornman et al., 2012, Laursen & Frikke, 2013).
Such a geographical redistribution might be due to birds responding to milder winters by shifting to new areas with environmental conditions, which were previously too severe, but are now tolerable (Maclean et al., 2008). Additionally, migration flights are costly, so a reduction of distance to breeding sites probably saves energy (Fox et al., 2015).
To keep trace with the optimal breeding conditions in combination with the even faster changing northern climate, bird species are moving northwards (Lehikoinen & Virkkala, 2016). For example, the spoonbill (Platalea leucorodia) and the Mediterranean gull (Larus cachinnans), together with other species that formerly occurred in southern Europe, have become regular breeders in the Wadden Sea (Koffijberg et al., 2015).
For birds, climate change is not only a matter of temperature increase, but also change in precipitation regime. Southern European and more southern wetlands become smaller or even disappear due to reduced rainfall. This severely affects wintering conditions for some bird populations, which use the Wadden Sea on their migratory route (Fox et al., 2015). As a result, water birds formerly relying on these sites may shift to other regions or might face decreasing population sizes when failing to adapt (Møller et al., 2008).
2.2.5 Shifts in timing of migration and reproduction
In the Wadden Sea more birds stay in the eastern part during mild winters and depart earlier to the breeding grounds when springs are warm (Bairlein & Exo, 2007). In addition, long-term changes trigger responses, although these temperature-mediated shifts are not necessarily similar in all bird species.
During the last 30 years, the greylag goose (Anser anser) departs three weeks earlier and lapwing (Vanellus vanellus) four weeks earlier than in former time, opposite to barnacle geese (Branta leucopsis) that have postponed the departure by four weeks, probably taking advantage of an early growth in vegetation (Laursen & Frikke, 2013). These examples indicate that species wintering in the Wadden Sea respond to climate change by developing different migration and stopover strategies.
In a common tern (Sterna hirundo) colony at the German North Sea coast, arrival date and body mass after spring migration was linked with environmental conditions including climate indices (Szostek et al., 2015). Because the phenological changes differed for birds of different age, common terns might be subject to differing environmental constraints or respond differently to conditions during their annual cycle depending on their age (Szostek et al., 2015).
Common seals (Phoca vitulina) give birth in the Wadden Sea area during the summer months. For the Dutch Wadden Sea, analyses of the number of pups as counted by means of annual aerial surveys showed an average advanced shift of 0.71 d yr-1 over the period 1974 and 2009 (Reijnders et al., 2010), whilst analysis of stranding dates of orphaned pups admitted for rehabilitation revealed an average shift of 0.88 days per year over the period 1974-2008 (Osinga et al., 2012).
The timing of common seal reproduction showed a similar shift in other parts of the Wadden Sea, with pups being born -0.68 d yr-1 in Schleswig Holstein and -0.59 d yr-1 in Niedersachsen (Reijnders et al., 2010). This shift in phenology is considered to reflect an adaptive response of the animals to altered local circumstances, amongst others food availability and climate change (Reijnders et al., 2010; Osinga et al., 2012).
2.2.6 Changes in trophic transfer and trophic interactions
An earlier seasonal onset of zooplankton grazing due to increased spring temperatures (Wiltshire & Manly, 2004) may restrain the peak in microalgal biomass during the spring phytoplankton bloom, and promote regenerative production by flagellates at the expense of diatoms. Within the North Sea, a recent change in temperature has caused modified trophic interactions in the food chain, for example, the trophic transfer from phytoplankton via zooplankton (Calanus finmarchicus) to cod (Kirby & Beaugrand, 2009). Subsequently, sea birds depending on fish abundance, need to shift towards more preferable conditions or decline in number (Zhang et al., 2015).
As the spring migration of predatory shrimps and crab species is enhanced by warmer conditions, the resulting increase in predation pressure may reduce the survival of their prey, including Baltic tellins (Macoma balthica) (Philippart et al., 2003; Beukema & Dekker, 2005; Beukema et al., 2009). Such bivalve species may, however, adapt to these conditions by advancing their timing of spawning and thereby escaping the enhanced predation pressure (Philippart et al. 2014). This may, amongst other factors including reduced fishing efforts, have contributed to the recent large recruitment events of Baltic Tellins in the Dutch Wadden Sea (Compton et al. 2016).
Temperature also affects population size in birds indirectly through the food web. As the lack of cold winters increases predatory pressure on blue mussel (Mytilus edulis) spat, mussel stocks decrease (Beukema, 2015) negatively affecting higher trophic levels, such as common eiders (Somateria mollissima).
Further, decreasing numbers of eiders breeding in the large Baltic colony at Christiansø, east of Bornholm, and wintering in the Wadden Sea (from ~3,000 pairs in 1992 to ~1,000 pairs in 2008), is highly related to the mussel stocks in the Wadden Sea (Laursen & Møller, 2013). Moreover, in the Dutch part of the Wadden Sea, the decrease in the contribution of cultured mussels to the diet of Eiders during the course of winter seasons, indicates that harvesting of mussels from the culture plots might further reduce this high-quality food source, forcing the eiders to switch to less profitable prey at the end of the winter, which has reproductive consequences (Laursen & Møller, 2013; Cervencl et al., 2015).
Recently, evidence was presented for a case of such body shrinkage, potentially due to malnutrition in early life. A long-distance migratory bird (red knot Calidris c. canutus) experiences high warming rates at its Arctic breeding grounds, produces smaller offspring with shorter bills during summers with early snowmelt. The short-billed individuals showed reduced survival on their tropical wintering grounds, probably because they could no longer get access to deeply buried and most profitable food sources. This finding illustrates the consequences of climate change during juvenile growth at breeding grounds for survival at later stages during the life cycle (Van Gils et al., 2016).
2.3 Other effects of climate change
2.3.1 Diseases and parasites
Disease has been implicated in the recent increase in occurrence and severity of mass mortalities, especially in marine invertebrates (Fey et al., 2015). While disease associated mortalities also occur in Wadden Sea species, cases where disease could be convincingly linked to these phenomena have been rare. Notable exceptions include disease associated mass mortalities of European harbour seals Phoca vitulina as the result of a phocine distemper virus in 1988 and 2002 (Härkönen et al., 2006) and Pacific oysters Crassostrea gigas in 2005 (Watermann et al., 2008).
Water temperature is positively correlated with the development of many disease agents including various infective Vibrio bacteria (Wendling and Wegner, 2013) as well as many parasites, thereby increasing the risk of infection and diseases during summer (Schade et al., 2015). In the future, warmer summers are expected to occur more often, favouring growth of Vibrio spp. that could also be infective for humans (Sterk et al., 2015).
Interannual temperature fluctuations are likely to alter host–parasite interactions, e.g., via common small fish species such as three-spined stickleback Gasterosteus aculeatus, Atlantic herring Clupea harengus, European sprat Sprattus sprattus and lesser sand eel Ammodytes tobianus (Schade et al., 2015). Different phenological responses of hosts and parasites will also lead to mismatches between coevolved species pairs and might force parasites to include other species into their life cycle.
Similarly, temperature dependent predation interactions can affect host-parasite interactions, when predators prey on free-living stages of parasites (Goedknegt et al., 2015). Since parasitism and disease can have profound impacts on sensitive ecosystems with direct and indirect effects mediating disease risk under climate change, similar considerations should be taken into account as for other species interactions.
2.3.2 Synergistic and large-scale effects
Furthermore, other human pressures, such as fisheries, pollution and hydrodynamics, may interact synergistically with climate change effects. For example, Atlantic cod has an optimal thermal niche of 9 °C to 10°C and is equal to the thermal niche of zooplankton, which serves as main diet for cod recruitment. Pressure of fisheries initially affects the abundance of cod, though a steady decline is caused by the decreased abundance of zooplankton, as a function of increased temperature. So, climate change indirectly drives cod to the edge of its thermal niche and makes it a highly vulnerable species (Beaugrand & Kirby, 2010).
Several NW European seas, including the North Sea, the Baltic Sea, the Black Sea and the Mediterranean Sea, underwent a major change at the end of the 1980s that encompassed local atmospheric, hydrological, and ecological conditions (e.g., Edwards et al., 2002, Conversi et al., 2010, Ljunggren et al., 2010). These regime shifts appear to be linked to the larger scale, northern hemisphere climatic conditions. Such parallel developments bear implications for the development of climate change scenarios, as synchronous shifts may provide the key for distinguishing local (i.e., basin) anthropogenic drivers, such as eutrophication or fishing, from larger scale (hemispheric) climate drivers.
3. Recommendations
3.1 Monitoring
3.1.1 Safe-guarding relevant long-term field observations
Recommendation #1: Continuing and further strengthening long-term field observations (including TMAP), the scientific analyses of the data and the publication of the results.
In line with the expectations in the Wadden Sea Plan 2010 (CWSS, 2010), average temperatures in the Wadden Sea have increased further as a result of global warming. Since 2009, impacts of climate change on Wadden Sea habitats and species became more evident, including shifts in species composition and phenology. These findings stress the importance of continuous and consistent monitoring efforts at a trilateral scale. Given the ecosystem dynamics within the Wadden Sea, a.o. as the result of climate change, annual reports on the status of this area are recommended.
3.1.2 Efficient increase of spatiotemporal resolution
Recommendation #2: Making more intense use of innovative techniques, including satellite images and automated sensor networks, to increase the temporal and spatial resolution without increasing costs at a similar magnitude.
Shifts in phenology have been recently observed for many taxonomic groups, including plankton, benthos, fish, birds and sea mammals in the Wadden Sea and the adjacent North Sea. Species-specific changes in phenology underline that different species (and even different life phases within one species) might be subject to differing environmental constraints and subsequently respond differently to environmental changes during their life.
Annual sampling campaigns are not sufficient to capture shifts in species-specific phenology. Furthermore, the sensitivity of habitats and species to climate change appears to be related to (other) environmental conditions, which vary throughout the Wadden Sea (e.g., winter temperatures, tidal amplitudes). Hindcasting and forecasting impacts of climate change, therefore, requires information on high spatial and temporal resolutions for the different functional groups.
Such information can only be gathered by deploying new techniques which are able to capture higher resolution in time (automated networks), space (satellite images) and biodiversity (e.g., molecular metabarcoding, eDNA).
3.1.3 Addressing appropriate scales in time and space
Recommendation #3: Performing monitoring at larger than local scales, i.e. including the potential sources and sinks, in order to understand the origin and fate of Wadden Sea organisms as the result of climate change.
Observed shifts in distribution for species within many taxonomic groups (e.g., plankton, macrozoobenthos, fish, birds and sea mammals) might be related to changes in local circumstances or due to large-scale shifts in environmental conditions, including climate change. On average, so far more southern species enter the Wadden Sea than northern species migrate out, which resulted in an overall increase in biodiversity. To better understand (and possibly forecast) such shifts in distribution, information on shifts in distribution of coastal organisms at a larger scale incorporating adjacent regions (e.g., North Sea) should be gathered and included.
3.2 Research
3.2.1 Determining dose-effect relationships
Recommendation #4: Gathering more information on dose-effect relationships, preferably within one scientific framework (e.g., Dynamic Energy Budgets), and on probabilities of occurrences of specific environmental conditions at relevant spatial scales (i.e., from ecotopes via habitats to tidal basins).
Various aspects of weather and climate (including temperature, sea level change, wind and rainfall) are expected to further affect species and habitats of the Wadden Sea. The effects depend on the nature of the environmental changes (e.g., frequency and height of heat waves) and the resilience of the species or habitat to this change (e.g., tolerance to high temperatures).
Combining the local information on environmental change (e.g., SST), an organism’s ecophysiology (potential adaptation of an organism's physiology to environmental conditions) and ecological processes (e.g., rates of mineralisation, primary production, filtration, parasite transmission) is essential to transform observational monitoring data into mechanistic predictive modelling capacity of the impacts of climate change on Wadden Sea communities.
3.2.2 Determining synergistic effects
Recommendation #5: Implementing an existing (or developing a new) tool to weigh separate and synergistic effects of climate change on Wadden Sea species and habitats.
With regard to the effects of climate change on species and habitats, a distinction can be made of (i) the effect of one factor (e.g., sea level rise) on one species (e.g., mussels) or habitat (tidal flats), (ii) the effect of a combination of factors (e.g., sea level rise and SST) on one species or habitat, and (iii) the interactive nature of a combination of factors on a suite of species and habitats. The interactive effects of climate change parameters need to be quantified accurately and can then be used to identify key parameters.
Indicators of the status of an ecosystem are most useful if they are sufficiently comprehensive to provide information about the status of and potential interactions among all components of this ecosystem (Halpern et al., 2012). Applying an existing tool (e.g., the Ocean Health Index) or developing a new tool (in case the existing tools are considered to be inappropriate) would also allow for annual reports of the status of the Wadden Sea and for exploring the consequences of various scenarios of climate change (e.g., KNMI, 2014) on different spatial scales to identify vulnerable regions and habitats within these.
Preferably, the tool should incorporate all relevant aspects of the Wadden Sea area, i.e., next to abiotic and biotic components also economic and socio-culturally valued aspects (Philippart et al., 2014b).
3.3 Management
3.3.1 Trilateral adaptive management approach
Recommendation #6: Developing a trilateral adaptive management procedure for the protection of species, habitats and sites for ensuring their value for future generations.
Research and monitoring are key components for informed policy and decision making, especially when coastal management aims to achieve a sustainable balance between protecting nature and allowing multiple human uses. However, an important challenge is to evaluate, integrate, and extend existing monitoring systems to provide a more effective and less biased platform for decision-making toward sustainability.
Ensuring that the resource exploitation of the Wadden Sea is sustainable and minimizes the effects on species and habitats within the dynamics and uncertainties set by climate change requires a continuous feed-back of targeted information on the status of the Wadden Sea ecosystem to decision makers. Such information could, for example, be based upon results of key predictions for (environmental) conditions where management can have the highest beneficial impact to achieve their goals. This information can then be used to evaluate past actions and consider the effectiveness of future management regulations.
4. Summary
During the last decades, the Wadden Sea ecosystem was already found to show clear signs of recent climate change, including temperature increase, import of southern warm-water species and changes in the timing of life cycle events. In this report, an update of the 2009 QSR is given on the most recent status and trends of direct (a.o. temperature, sea level, tidal amplitudes, wind, precipitation) and indirect impacts of climate change on key elements of the Wadden Sea ecosystem. The historically observed indirect impacts were found to have increased with additional species’ shifts in geographical distributions (e.g., eel pout, sea bass, Eurasian curlew, common eider) and in the timing of migration (e.g., greylag goose, lapwing) and reproduction (e.g., common seals).
New findings on impacts of climate change on Wadden Sea species included the reduced survival of young migratory birds (red knot) due to changing food availability at their Arctic breeding grounds. Based on the implications of such findings for conservation targets, it is recommended to safe-guard and strengthen existing long-term field observations, including a cost-effective increase in spatio-temporal resolutions. To translate these observational monitoring data into mechanistic predictive modelling capacity of the impacts of climate change on Wadden Sea communities, knowledge gaps with respect to dose-effect relationships and synergistic effects should be filled. To ensure that human activities within the Wadden Sea are sustainable (with minimum effects on species and habitats within the dynamics and uncertainties set by ongoing climate change), a continuous feed-back of targeted information on the status of the Wadden Sea ecosystem to decision makers is required, e.g., by means of an interactive open-access data and information platform for the Trilateral Wadden Sea.
About the authorsC.J.M. Philippart1,2,3, L. Mekkes3,4, C. Buschbaum5, K.M. Wegner5, K. Laursen6 1 NIOZ Royal Netherlands Institute of Sea Research & Utrecht University, Department of Coastal Systems, P.O. Box 59, 1790 AB Den Burg (Texel), NL 2 Wadden Academy, Ruiterskwartier 121A, 8911 BS Leeuwarden, NL 3 Utrecht University, Department of Physical Geography, Coastal Processes, P.O. Box 80.115, 3508 TC Utrecht, NL 4 University of Amsterdam, Institute for Biodiversity and Ecosystem Dynamics, University of Amsterdam, P.O. Box 94248, 1090 GE, Amsterdam, NL 5 AWI Alfred Wegener Institute for Polar- and Marine Research, Coastal Ecology Wadden Sea Station Sylt, Hafenstrasse 43, 25992 List (Sylt), DE 6 University of Aarhus, Department of Bioscience, Wildlife Ecology, Grenåvej 14, 8410 Rønde, DK |
References
Alexandersson, H., H. Tuomenvirta, T. Schmith & K. Iden (2000) Trends of storms in NW Europe derived from an updated pressure data set. Climate Research 14: 71–73.
Alheit, J. & E. Hagen (1997) Long-term climate forcing of European herring and sardine populations. Fisheries Oceanography 6: 130–139.
Bairlein, F. & K.M. Exo (2007) Climate Change and Migratory Birds. In: Reineking, B., and Südbeck, P. (Eds.). Seriously Declining Trends in Migratory Waterbirds: Causes-Concerns- Consequences. Proceedings of the International Workshop on 31 August 2006 in Wilhelmshaven, Germany. Wadden Sea Ecosystem No. 23. Common Wadden Sea Secretariat, Wilhelmshaven: 43-52.
Bartholdy, A.T., J. Bartholdy & A. Kroon (2010) Salt marsh stability and patterns of sedimentation across a backbarrier platform. Marine Geology 278: 31-42.
Bartholomä, A. & B. Flemming (2007) Progressive grain-size sorting along an intertidal energy gradient. Sedimentary Geology 20: 464-472.
Beaugrand, G., X. Harlay & M. Edwards (2014) Detecting Plankton Shifts in the North Sea: A New Abrupt Ecosystem Shift between 1996 and 2003. Marine Ecology Progress Series 502: 85–104.
Beaugrand, G (2004) The North Sea regime shift: Evidence, causes, mechanisms and consequences. Progress in Oceanography 60: 245–262
Beaugrand, G., K. M. Brander, J. Lindley, S. Souissi & P. C. Reid (2003) Plankton effect on cod recruitment in the North Sea. Nature 426: 661-664.
Beaugrand, G., & R.R. Kirby (2010) Climate, plankton and cod. Global Change Biology 16: 1268–1280.
Beare, D., F. Burns, E. Jones, K. Peach, E. Portilla, T. Greig, E. Mckenzie & D. Reid (2004) An increase in the abundance of anchovies and sardines in the north-western North Sea since 1995. Global Change Biology 1: 1209–1213.
Belkin, I.M (2009) Rapid warming of large marine ecosystems. Progress in Oceanography 81: 223-236.
Beukema, J.J., W.J. Wolff, J.J.W.M. Brouns (Eds.) (1990) Expected Effects of Climatic Change on Marine Coastal Ecosystems. Kluwer Academic Publishers, Dordrecht, 221 pp.
Beukema, J.J. (1992) Expected changes in the Wadden Sea benthos in a warmer world: lessons from periods with mild winters. Netherlands Journal of Sea Research 30: 73-79.
Beukema, J.J. & R. Dekker (2005) Decline of recruitment success of cockles and other bivalves in the Dutch Wadden Sea: possible role of climate change, predation on postlarvae and fisheries. Marine Ecology Progress Series 287: 149-167.
Beukema, J.J., R. Dekker & J.M. Jansen (2009) Some like it cold: populations of the tellinid bivalve Macoma balthica (L.) suffer in various ways from a warming climate. Marine Ecology Progress Series 384: 135-145.
Beukema, J. J. & R. Dekker (2011) Increasing species richness of the macrozoobenthic fauna on tidal flats of the Wadden Sea by local range expansion and invasion of exotic species. Helgoland Marine Research 65: 155-164.
Beukema, J. J., R. Dekker, M.R. van Stralen & J. de Vlas (2015) Large-scale synchronization of annual recruitment success and stock size in Wadden Sea populations of the mussel Mytilus edulis. Helgoland Marine Research 69: 327–333
Boersma M., N. Grüner, T.N. Signorelli, P.E. Montoro González, M.A. Peck & K.H. Wiltshire (2016) Projecting effects of climate change on marine systems: is the mean all that matters? Proceedings of the Royal Society B 283: 20152274.
Boonstra, M., Y. Radstake, K. Rebel, G. Aarts & C.J. Camphuysen (2013) Harbour porpoises (Phocoena phocoena) in the Marsdiep area, the Netherlands: new investigations in a historical study area. Lutra 5: 59-71.
Brander, K (2007) Global fish production and climate change. Proceedings of the National Academy of Sciences of the USA 104: 19709–19714.
Burchard, H., & R.D Hetland (2010) Quantifying the contributions of tidal straining and gravitational circulation to residual circulation in periodically stratified tidal estuaries. Journal of Physical Oceanography 40: 1243-1262.
Buschbaum, C., D. Lackschewitz & K. Reise (2012) Nonnative macrobenthos in the Wadden Sea ecosystem. Ocean & Coastal Management 68: 89-101
Camphuysen, C.J., C.M. Berrevoets, H.J.W.M. Cremers, A. Dekinga, R. Dekker, B.J. Ens, T.M. van der Have, R.K.H. Kats, T. Kuiken, M.F. Leopold, J. van der Meer & T. Piersma (2002) Mass mortality of common eiders (Somateria mollissima) in the Dutch Wadden Sea, winter 1999/2000: starvation in a commercially exploited wetland of international importance. Biological Conservation 106: 303–317
Cervencl, A., K. Troost, E. Dijkman, M. de Jong, C. Smit, M.F. Leopold & B.J. Ens (2015) Distribution of wintering Common Eider Somateria mollissima in the Dutch Wadden Sea in relation to available food stocks. Marine Biology 162: 153-168.
Chen, I.C., Hill, J.K., Ohlemuller, R., Roy, D.B. & Thomas, C.D (2011) Rapid range shifts of species associated with high levels of climate warming. Science 333: 1024-1026.
Conversi, A., S.F. Umani, T. Peluso, J.C. Molinero, A. Santojanni & M. Edwards (2010) The Mediterranean Sea regime shift at the end of the 1980s, and intriguing parallelisms with other European basins. PLoS One 5: e10633.
Common Wadden Sea Secretariat (CWSS) (2008) Nomination of the Dutch-German Wadden Sea as a world heritage site. In: World Heritage Nomination Project Group, Wadden Sea Ecosystem, vol. 24. Common Wadden Sea Secretariat, Wilhelmshaven, Germany. 1-200.
Compton, T.J., Bodnar W, Koolhaas A, Dekinga A, Holthuijsen S, ten Horn J, McSweeney N, van Gils JA and Piersma T (2016) Burrowing Behavior of a Deposit Feeding Bivalve Predicts Change in Intertidal Ecosystem State. Frontiers in Ecology and Evolution 4:19. doi: 10.3389/fevo.2016.00019
De Winter, R. C., A. Sterl, J. W. de Vries, S. L. Weber & B. G. Ruessink (2012) The effect of climate change on extreme waves in front of the Dutch coast. Ocean Dynamics 62: 1139–1152.
Dolch, T. & K. Reise (2010) Long-term displacement of intertidal seagrass and mussel beds by expanding large sandy bedforms in the northern Wadden Sea. Journal of Sea Research 63: 93-101.
Donker, J.J.A., M. van der Vegt & P. Hoekstra (2014) Wave forcing in the Dutch Wadden Sea: effects on mussel habitats. PhD thesis. University of Utrecht, Utrecht.
Edwards, M., G. Beaugrand, P.C. Reid, A.A. Rowden & M.B. Jones (2002) Ocean climate anomalies and the ecology of the North Sea. Marine Ecology Progress Series 239: 1-10.
Edwards, M., & A.J. Richardson (2004) Impact of climate change on marine pelagic phenology and trophic mismatch. Nature 363: 2002–2005.
Elias, E. P. L., A.J.F. van der Spek, Z.B. Wang & J. de Ronde (2012) Morphodynamic development and sediment budget of the Dutch Wadden Sea over the last century. Netherlands Journal of Geosciences 91: 293-310.
Engelhard G.H., D.A. Righton & J.K., Pinnegar (2014) Climate change and fishing: a century of shifting distribution in North Sea cod. Global Change Biology 20: 2473-2483.
Feser, F., M. Barcikowska, O. Krueger, F. Schenk, R. Weisse, L. Xia (2015) Storminess over the North Atlantic and Northwestern Europe − a review. Quarterly Journal of the Royal Meteorological Society 141: 350-382.
Flöser, G., H. Burchard & R. Riethmüller (2011) Observational evidence for estuarine circulation in the German Wadden Sea. Continental Shelf Research 31: 1633-1639.
Folmer, E. O., J. Drent, K. Troost, H. Büttger, N. Dankers, J. Jansen, M. Van Stralen, G. Millat, M. Herlyn And C. J. M. Philippart (2014) Large-scale spatial dynamics of intertidal mussel (Mytilus edulis) bed coverage in the German and Dutch Wadden Sea. Ecosystems 17: 550–566.
Fox, D.F., J.E. Jónsson, T. Aarvak, T. Bregnballe, T.K. Christensen, K. K. Clausen, P. Clausen, L. Dalby, T.E. Holm, D. Pavón-Jordan, K. Laursen, A. Lehikoinen, S.-H. Lorentsen, A.P. Møller, M. Nordström, M. Öst, P. Söderquist, O.R. Therkildsen (2015) Current and potential threats to Nordic duck populations – a horizon scanning exercise. Annales Zoologici Fennici 52: 193-220.
Genner, M.J., D.W. Sims, A.J. Southward, G.C. Budd, P. Masterson, M. McHugh ... & S.J. Hawkins (2010) Body size‐dependent responses of a marine fish assemblage to climate change and fishing over a century‐long scale. Global Change Biology 16: 517-527.
Gibbons, M.J. & A.J. Richardson (2009) Patterns of jellyfish abundance in the North Atlantic. Hydrobiologia 616: 51–65.
Goedknegt, M.A., J.E. Welsh, J. Drent & D.W. Thieltges (2015) Climate change and parasite transmission: how temperature affects parasite infectivity via predation on infective stages. Ecosphere 6: 96.
Halpern, B.S., C. Longo, D. Hardy, K.L. McLeod, J.F. Samhouri, S.K. Katona, ... & A.A. Rosenberg (2012) An index to assess the health and benefits of the global ocean. Nature 488: 615-620.
Hammond, P.S., K. Macleod, P. Berggren, D.L. Borchers, L. Burt, A. Caladas & J.A. Vazquez (2013) Cetacean abundance and distribution in European Atlantic shelf waters to inform conservation and management. Biological Conservation 164: 107-122.
Hiddink, J.G., M.T. Burrows & J. García Molinos (2015) Temperature tracking by North Sea benthic invertebrates in response to climate change. Global Change Biology 21: 117-129.
Hiddink, J.G. & R. ter Hofstede (2008) Climate induced increases in species richness of marine fishes. Global Change Biology 14, 453-460.
Hellmann, G. (1917) Überstrenge Winter. Abhandlungen der Königlich Preussische Akademie der Wissenschaften. Reichsdruckerei, Berlin.
Hofstede, J.L (2015) Theoretical considerations on how Wadden Sea tidal basins may react to accelerated sea level rise. Zeitschrift für Geomorphologie 59, 377-391.
Hornman, M., M. van Roomen, F. Hustings, K. Koffijberg, E. van Winden, L. Soldaat (2012) Populatietrends van overwinterende en doortrekkende watervogels in Nederland in 1975-201. Limosa 85: 97-116.
IPCC (2007) Climate Change 2007: Impacts, Adaptation and Vulnerability. Contribution of Working Group II to the Fourth Assessment Report of the Intergovernmental Panel on Climate Change, M.L. Parry, O.F. Canziani, J.P. Palutikof, P.J. van der Linden and C.E. Hanson, Eds., Cambridge University Press, Cambridge, UK, 976 pp.
IPCC (2013) Climate Change 2013: The Physical Science Basis. Contribution of Working Group I to the Fifth Assessment Report of the Intergovernmental Panel on Climate Change [Stocker, T.F., D. Qin, G.-K. Plattner, M. Tignor, S.K. Allen, J. Boschung, A. Nauels, Y. Xia, V. Bex and P.M. Midgley (eds.)]. Cambridge University Press, Cambridge, United Kingdom and New York, NY, USA, 1535 pp.
Joint Monitoring Group of Breeding Birds (JMGBB) (2013) CWSS Workshop Report Breeding Birds in Trouble, Wilhelmshaven, 18 April 2013.
Jung, A.S. A.G. Brinkman, E.O. Folmer, P.M.J. Herman, H.W. van der Veer, C.J.M. Philippart (2017) Long-term trends in nutrient budgets of the western Dutch Wadden Sea (1976-2012). Journal of Sea Research, Available online, dx.doi.org/10.1016/j.seares.2017.02.007.
Kirby, R.R., G. Beaugrand (2009) Trophic amplification of climate warming. Proceedings of the Royal Society B 276: 4095–4103.
Koffijberg, K., K. Laursen, B. Hälterlein, G. Reichert, J. Frikke & L. Soldaat (2015) Trends of Breeding Birds in the Wadden Sea 1991 - 2013. Wadden Sea Ecosystem No. 35. Common Wadden Sea Secretariat, Joint Monitoring Group of Breeding Birds in the Wadden Sea, Wilhelmshaven, Germany.
Kristensen, I. (1958) Differences in density and growth in a cockle population in the Dutch Wadden Sea. Arch. Néerl. Zool. 12, 351-453.
Lambert, E., G.J. Pierce, K. Hall, T. Brereton, T.E. Dunn, D. Wall, P.D. Jepson, R. Deauville & C.D. MacLeod (2014) Cetacean range and climate in the eastern North Atlantic: future predictions and implications for conservation. Global Change Biology 20: 1782-1793.
Laursen, K., K. Asferg, J. Frikke & P. Sunde (2009) Mussel fishery affects diet and reduce body condition of eider Somateria mollissima in the Wadden Sea. Journal of Sea Research 62: 22-30.
Laursen, K., P.S. Kristensen & P. Clausen (2010a) Assessment of blue mussel Mytilus edulis fisheries and waterbird shellfish-predator management in the Danish Wadden Sea. Ambio 39: 476-485.
Laursen, K., J. Blew, K. Eskildsen, K. Gunther, B. Halterlein, R. Kleefstra, G. Luersen, P. Potel & S. Schrader (2010b) Migratory waterbirds in the Wadden Sea 1987-2008. In: Wadden Sea Ecosystem, vol. 30. Common Wadden Sea Secretariat, Wilhelmshaven, Germany, pp. 1-170.
Laursen, K. & J. Frikke (2013) Staging waterbirds in the Danish Wadden Sea 1980-2010. Dansk Orn. Foren. Tidsskr. 107: 1-184.
Laursen, K. & A.P. Møller (2014) Long-Term Changes in Nutrients and Mussel Stocks Are Related to Numbers of Breeding Eiders Somateria mollissima at a large Baltic Colony. PLoS One 9 (4): e95851.
Lehikoinen, A. & R. Virkkala (2016) North by north-west: Climate change and directions of density shifts in birds. Global Change Biology 22: 1121–1129.
Ljunggren, L., A. Sandström, U. Bergström, J. Mattila, A. Lappalainen, G. Johansson & B.K. Eriksson (2010) Recruitment failure of coastal predatory fish in the Baltic Sea coincident with an offshore ecosystem regime shift. ICES Journal of Marine Science: Journal du Conseil, 109.
Luczak, C., G. Beaugrand, J.A. Lindley, J.M. Dewarumez, P.J. Dubois & R.R. Kirby (2012) North Sea ecosystem change from swimming crabs to seagulls. Biological Letters 8: 821−824.
Maclean, I.M.D., G.E. Austin, M.M. Rehfisch, J. Blew, O. Crowe, S. Delany, K. Devos, B. Deceuninck, K. Gunther, K. Laursen, M. Van Roomen & J. Wahl (2008) Climate change causes rapid changes in the distribution and site abundance of birds in winter. Global Change Biology 14: 2489-2500.
Madsen, A.T., A.S. Murray, T.J. Andersen & M. Pejrup (2007) Temporal changes of accretion rates on an estuarine salt marsh during the late Holocene –Reflection of local sea level changes? The Wadden Sea, Denmark. Marine Geology 242: 221-233.
Meehl, G., T.F. Stocker, W.D. Collins, P. Friedlingstein, A.T. Gaye, J.M. Gregory,A. Kitoh, R. Knutti, J.M. Murphy, A. Noda, S.C.B, Raper, I.G. Watterson, J. Weaver & Z.C. Zhao (2007) Global climate projections. In: S. Solomon, D. Qin, M. Manning, Z. Chen, M. Marquis, K.B., Averyt, M. Tignor & H.L. Mille (eds): Climate Change 2007: The Physical Science Basis. Contribution of Working Group 1 to the Fourth Assessment Report of the Intergovernmental Panel on Climate Change. Cambridge. University Press (Cambridge, UK and New York, USA), 996 pp.
Mieszkowska, N., R. Leaper, P. Moore, M.A. Kendall, M.T. Burrows, D. Lear, E. Poloczanska, K. Hiscock, P.S. Moschella, R.C. Thompson, R.J. Herbert, D. Laffoley, J. Baxter, A.J. Southward & S.J. Hawkins (2005) Marine biodiversity and climate change: assessing and predicting the influence of climatic change using intertidal rocky shore biota. Occup. Pub. J. Mar. Biol. Assoc. UK 20: 53 pp.
Møller, A.P., D. Rubolini & E. Lehikoinen (2008) Populations of migratory bird species that did not show a phonological response to climate change are declining. Proceedings of the National Academy of Science of the United States of America 105: 16195-19200.
Munaretto, S. & J.E.M. Klostermann (2011) Assessing adaptive capacity of institutions to climate change: A comparative case study of the Dutch Wadden Sea and the Venice Lagoon. Climate Law 2: 219–250.
Nauw, J., C.J.M. Philippart, B.J. Ens, M. Duran-Matute & T. Gerkema (2016) Estimates of exposure times in the Wadden Sea: a comparison of methods. Manuscript submitted to the Journal of Sea Research.
Oost, A., P. Kabat, A. Wiersma & J. Hofstede (2009) Climate change. In: Marencic, H., De Vlas, J. (Eds.), Thematic Report No. 4.1. Quality Status Report 2009. Wadden Sea Ecosystem, vol. 25. Common Wadden Sea Secretariat, Trilateral Monitoring and Assessment Group, Wilhelmshaven, Germany.
Osinga, N., I. Pen, H.A.U. De Haes & P.M. Brakefield (2012) Evidence for a progressively earlier pupping season of the common seal (Phoca vitulina) in the Wadden Sea. Journal of the marine biological Association of the United Kingdom 92: 1663-1668.
Parmesan, C. & G. Yohe (2003) A globally coherent fingerprint of climate change impacts across natural systems. Nature 421: 37-42.
Perry, A.L., P.J. Low, J.R. Ellis & J.D. Reynolds (2005) Climate change and distribution shifts in marine fishes. Science 30: 1912–1915.
Peschko, V., K. Ronnenberg, U. Siebert & A. Gilles (2016) Trends of harbour porpoise (Phocoena phocoena) density in the southern North Sea. Ecological Indicators 60: 174-183.
Philippart, C. J.M., H.M. van Aken, J.J. Beukema, O.G. Bos, G.C. Cadée & R. Dekker (2003) Climate‐related changes in recruitment of the bivalve Macoma balthica. Limnology and Oceanography 48: 2171-2185.
Philippart, C.J.M. & E. Epping (2009) Climate change and ecology. Thematic Report No. 4.2. In: Marencic, H., De Vlas, J. (Eds.) (2009) Quality Status Report 2009. Wadden Sea Ecosystem, vol. 25. Common Wadden Sea Secretariat, Trilateral Monitoring and Assessment Group, Wilhelmshaven, Germany.
Philippart, C.J.M., R. Anadón, R. Danovaro, J.W. Dippner, K.F. Drinkwater, S.J. Hawkins, T. Oguz, G. O’Sullivan& P.C. Reid (2011) Impacts of climate change on European marine ecosystems: observations, expectations and indicators. Journal of Experimental Marine Biology and Ecology 400: 52-69.
Philippart, C.J.M., J.D.L. van Bleijswijk, J.C. Kromkamp, A.F. Zuur & P.M.J. Herman (2014a) Reproductive phenology of coastal marine bivalves in a seasonal environment. Journal of Plankton Research 36, 1512–1527.
Philippart, C.J.M., J. van Dijk & J. Enemark (2014b) Wadden monitoring in the spotlight: Cross-bordering maps on ecology and socio-economy of Denmark, Germany and The Netherlands. Wadden Sea Long-Term Ecosystem Research (WaLTER), Waddenacademie KNAW & Common Wadden Sea Secretariat (CWSS), 71 pp.
Poloczanska E.S., C.J. Brown, W.J. Sydeman, et al (2013) Global imprint of climate change on marine life. Nature Climate Change, 3: 919–925.
Reijnders, P. J., S.M. Brasseur & E.H. Meesters (2010) Earlier pupping in harbour seals, Phoca vitulina. Biology Letters 6: 854-857.
Reise,K., M. Baptist, P. Burbridge, N. Dankers, L. Fischer, B. Flemming, A.P. Oost & C. Smit (2010) The Wadden Sea – A Universally Outstanding Tidal Wetland. Wadden Sea Ecosystem No. 29. Common Wadden Sea Secretariat, Wilhelmshaven, Germany, 7-24.
Rindorf, A. & P. Lewy (2006) Warm, windy winters drive cod north and homing of spawners keeps them there. Journal of Applied Ecology 43: 445–453
Schade, F. M., M.J. Raupach & M. Wegner (2015) Seasonal variation in parasite infection patterns of marine fish species from the Northern Wadden Sea in relation to interannual temperature fluctuations. Journal of Sea Research (in press).
Schlüter, M. H., A. Merico, M. Reginatto, M. Boersma, K.H. Wiltshire & W.U.L.F. Greve (2010) Phenological shifts of three interacting zooplankton groups in relation to climate change. Global Change Biology 16: 3144–3153.
Sheridan, J.A. & D. Bickford (2011) Shrinking body size as an ecological response to climate change. Nature Climate Change 1: 401-406.
Seebens H., M.T. Gastner & B. Blasius (2013) The risk of marine bioinvasion caused by global shipping. Ecology Letters 1: 782-790.
Shamoun-Baranes, J. & C.J. Camphuysen (2013) Population dynamics in lesser black-backed gulls in the Netherlands reveal no response to North Sea regime shift: comment on Luczak et al (2012. Biol Lett 9: 20121085.
Sterk, A., F.M. Schets, A.M. de Roda Husman, T. de Nijs & J.F. Schijven (2015) Effect of Climate Change on the Concentration and Associated Risks of Vibrio Spp. in Dutch Recreational Waters. Risk Analysis, 35: 1717–1729.
Strasser, M., R. Dekker, K. Essink, C.P. Günther, S. Jaklin, I. Kröncke, P.B. Madsen, H. Michaelis & G. Vedel (2003) How predictable is high bivalve recruitment in the Wadden Sea after a severe winter. Journal of Sea Research 49: 47–57.
Suchrow, S., N. Pohlmann, M. Stock, K. Jensen (2012) Long-term surface elevation changes in German North Sea salt marshes. Estuarine, Coastal and Shelf Science 98, 71-83.
Szostek, K.L., S. Bouwhuis & P.H. Becker (2015) Are arrival date and body mass after spring migration influenced by large-scale environmental factors in a migratory seabird? Frontiers in Ecology and Evolution 3:42.
Valle, M., G. Chust, A. del Campo, M.S. Wisz, S.M. Olsen, J.M. Garmendia & A. Borja (2014) Projecting future distribution of the seagrass Zostera noltii under global warming and sea level rise. Biological Conservation 170: 74-85.
Van Beusekom, J.E.E., C. Buschbaum, K. Reise (2012) Wadden Sea tidal basins and the mediating role of the North Sea in ecological processes: scaling up of management? Ocean & Coastal Management 68: 69-78.
Van de Pol, M., Y. Vindenes, B.E. Sæther, S. Engen, B.J. Ens, K. Oosterbeek & J.M. Tinbergen (2010) Effects of climate change and variability on population dynamics in a long‐lived shorebird. Ecology 91: 1192-1204
Van de Pol, M., B.J. Ens, D. Heg, L. Brouwer, J. Krol, M. Maier, K.M. Exo, K. Oosterbeek, T. Lok, C.M. Eising & K. Koffijberg (2010) Do changes in the frequency, magnitude and timing of extreme climatic events threaten the population viability of coastal birds? Journal of Applied Ecology 47: 720-730.
Van der Spek, A.J.F. & D.J. Beets (1992) Mid-Holocene evolution of a tidal basin in the western Netherlands; a model for future changes in the northern Netherlands under conditions of accelerated sea-level rise? Sedimentary Geology 80: 185-197.
Van Hal, R., K. Smits & A.D. Rijnsdorp (2010) How Climate Warming Impacts the Distribution and Abundance of Two Small Flatfish Species in the North Sea. Journal of Sea Research 64: 76–84.
Van Roomen, M., K. Laursen, C. van Turnhout, E. van Winden, J. Blew, K. Eskildsen & B.J. Ens (2012) Signals from the Wadden sea: Population declines dominate among waterbirds depending on intertidal mudflats. Ocean & Coastal Management 68: 79–88.
Van Walraven, L., R. Dapper, I. Tulp, J.IJ. Witte, H.W. van der Veer (2016) Long-term patterns in fish phenology in the western Dutch Wadden Sea in relation to climate change. http://www.rijkewaddenzee.nl/assets/pdf/dossiers/natuur-en-landschap/FishStrategyWalkerPRWwebsiteDEF.pdf
Van Wijnen, H.J. & J.P. Bakker (2001) Long-term surface elevation change in salt marshes: a prediction of marsh response to future sea-level rise. Estuarine, Coastal and Shelf Science 52: 381-390.
Vinagre, C., F.D. Santos, H.N. Cabral & M.J. Costa (2009) Impact of climate and hydrology on juvenile fish recruitment towards estuarine nursery grounds in the context of climate change. Estuarine, Coastal and Shelf Science 85: 479-486.
Visser, M.E., & C. Both (2005) Shifts in phenology due to global climate change: the need for a yardstick. Proceedings of the Royal Society of London B: Biological Sciences 272: 2561-2569.
Vugteveen, P., M.M. van Katwijk, E. Rouwette, H. Lenders & L. Hanssen (2015) Developing an effective adaptive monitoring network to support integrated coastal management in a multiuser nature reserve. Ecology and Society 20: 59
Walther, G.R (2010) Community and ecosystem responses to recent climate change. Philosophical Transactions of the Royal Society B 365, 2019-2024.
Wanless, S., M. Frederiksen, J. Walton & M.P. Harris (2009) Long-term changes in breeding phenology at two seabird colonies in the western North Sea. Ibis 151: 274–285.
Weisse, R., H. Von Storch, F. Feser (2005) Northeast Atlantic and North Sea storminess as simulated by a regional climate model during 1958-2001 and comparison with observations. Journal of Climate 18: 465-479.
Wiltshire, K.H. & B.F.J. Manly (2004) The warming trend at Helgoland Roads, North Sea: phytoplankton response. Helgoland Marine Research 58: 269-273.
Witte, S., C. Buschbaum, J.E.E. Beusekom, K. Reise (2010) Does climatic warming why an introduced barnacle finally takes over after a lag of more than 50 years? Biological Invasions 12: 3579-3589.
Zhang, N., O. Vedder, P.H. Becker, S. Bouwhuis (2015) Contrasting between- and within-individual trait effects on mortality risk in a long-lived seabird. Ecology 96: 71-79.
Websites: http://www.knmi.nl/klimatologie/lijsten/hellmann.html
This report should be cited as: Philippart C.H.M., Mekkes L., Buschbaum C., Wegner K.M. & Laursen K. (2017) Climate ecosystems. In: Wadden Sea Quality Status Report 2017. Eds.: Kloepper S. et al., Common Wadden Sea Secretariat, Wilhelmshaven, Germany. Last updated 21.12.2017. Downloaded DD.MM.YYYY. qsr.waddensea-worldheritage.org/reports/climate-ecosystems